Understanding next-generation LED and Solar Cell Materials using time-resolved spectroscopy
Our lab is interested in helping find new forms of abundant and affordable clean energy, crucial to tackling the climate crisis. Emerging materials such as semiconductor nanocrystals and organic semiconductors are considerably better at absorbing light than conventional crystalline silicon, requiring less material to create a working solar cell or LED. Yet these new materials form chaotic structures in devices requiring careful study to understand how the arrangement of molecules at the microscale influences overall device function and stability.
Specifically, we study charge transfer photophysics in organic semiconductors and thermalization and trapping in semiconductor nanocrystals using time-resolved fluorescence and time-resolved x-ray pump probe techniques.
Contact Ben via email or stop by BiHall 545 if you are interested in learning more about our work or joining the lab.
Current Projects
Time-resolved x-ray and electron diffraction of semiconductor nanocrystals
We study semiconductor nanocrystals and organic semiconductors using time-resolved structural probes. We do this using an ultrafast optical pump and x-ray or electron bunch probe at national user facilities at Argonne National Laboratory’s Advanced Photon Source and the SLAC MeV-UED ultrafast electron diffraction instrument. These techniques are analogous to stop-motion animation, where we take lots of fast photos (in this case diffraction images) and are able to reconstruct a movie of the structural evolution of a material after it absorbs light. We use this to watch what structural features lead to losses of energy to heat (nonradiative recombination/trapping) as well as productive pathways (solar energy generation).
Quantifying the impact of residual catalyst concentration on organic semiconductor photophysics
Using the Middlebury ICP-MS spectrometer we measure the concentration of residual catalyst from synthesis leftover in organic semiconductor materials following various amount of purification or after adding catalyst back into a material. We then measure the photophysical and electrical properties of the OSC as a function of residual catalyst concentration. These catalysts are metals and can impact the functionality and efficiency of organic semiconductor based devices.
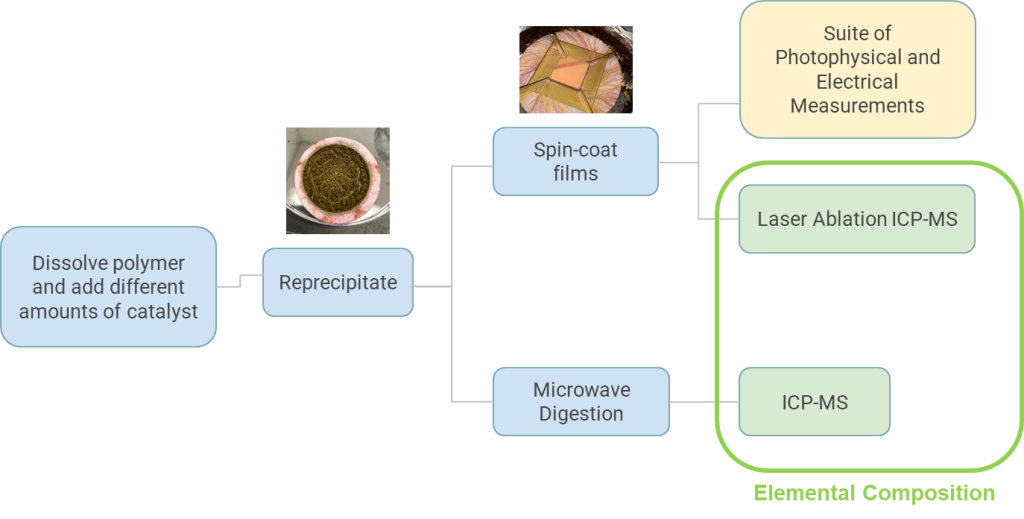
Charge transfer states in Organic Semiconductors
In photosynthesis, plants have evolved to facilitate a series of energy and charge transfer steps to drive excited electrons to a reaction center where the excited electron and hole are separated with high yield to eventually be stored as chemical energy (sugar). The step immediately prior to charge separation is charge transfer of the excited electron from a higher lying LUMO on a “donor” molecule to an adjacent “acceptor” molecule with a lower lying LUMO. A new metastable intermediate state named the charge transfer (CT) state is occupied at this step, with the excited electron on a reduced acceptor next to an oxidized donor molecule (D*A -> D+A– ). In the CT state, charges are particularly susceptible to nonradiative recombination (D+A– -> DA + heat), which must be avoided at all costs to maintain both stability and efficiency. Plants use precise positioning of molecules to further shuttle the charges apart from the CT state to final sites where desired redox reactions take place. In organic solar cells that target cost effective renewable energy, researchers work to replicate this tailored energy landscape with blended conjugated molecules to separate photoexcited charges and direct them to electrical contacts for collection and current flow.
We study how these charge transfer states in organic semiconductors can be altered and ultimately controlled by the presence of other nearby molecules to yield the best outcomes. To do this we use time-resolved photoluminescence and quantum yield spectroscopy techniques that measure how many light photons create energy that yields useful results.